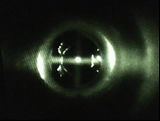
Ultracold atoms & molecules
Ultrafast lasers & ablation studies
Physics Research at Willamette University
Prof. Michaela Kleinert
Collins 314
Willamette University
Salem, OR 97301
Ultracold atoms and molecules
In a nutshell:
We are interested in studying the properties of ultracold atoms and molecules that have been formed from these ultracold atoms. At room temperature atoms move with several hundred or even thousand meters per second. That makes studying them in detail just as difficult as trying to make out the interior of a car on the highway. Fortunately, a clever combination of laser light and magnetic fields, the magneto-optical trap or MOT, can slow these atoms to speeds of just a few centimeters per second. This allows us to look at them more closely and study their properties in greater detail.
We are working with rubidium, an alkali-metal atom, and calcium, an alkaline-earth atom. Both species have been cooled and trapped individually in various labs across the world, but the combination of both atomic species together has not yet been studied. We are particularly interested in the study of collisions between these two different atomic species at ultracold and cold temperatures with the ultimate goal of creating cold molecules consisting of these two atoms. These molecules possess a magnetic and an electric dipole moment, making them excellent candidates for quantum information processing or studies of collisions in crossed electric and magnetic fields.
Student involvement:
Students of all levels, from Freshman to Senior, have the opportunity to be involved in every aspect of this state-of-the-art research project. They get exposed to lasers, atom-light interaction, ultra-high vacuum systems, electronics and computer control of a complex experiment. Individual projects vary depending on student interest and background. If you are interested in joining our research group please send an email to Prof. Kleinert [mkleiner AT willamette DOT edu].
The workhorse: The Magneto-Optical Trap (MOT):
The invention of the magneto-optical trap in 1987 [1], for which the Physics Noble Prize was awarded 10 years later [2], has been one of the major achievements in the field of atomic physics. It reduces the temperature of gaseous atoms from several hundred Kelvin to just a few 10-100 micro-Kelvin. This has lead to an explosion of high-precision spectroscopic data, the formation of Bose-Einstein Condensates and Fermi-degenerate gases, the creation of the atom laser and the formation of ultracold homo- and heteronuclear molecules, to name just a few. While initially only alkali-metal atoms were trapped due to their relatively simple level structure - they only contain a single electron in the valence shell -, by now several alkaline-earth, rare-earth metals and metastable gases have also been trapped. An up to date list of groups working with ultracold atoms and molecules can be found here.
So how does this trap work? For now let us assume a two-level atom, an atom that has only a ground state (this is the state in which the electron spends most of its time) and one excited state. If a photon of just the right amount of energy hits the atom it can be absorbed as shown below:
Note that while the absorption will always happen from the same direction (from the laser beam), the emission can happen in any direction (spontaneous emission). Thus, each time the atom absorbs a photon it feels a "kick" opposing the direction of propagation of the laser beam, and each time it emits a photon it feels a kick in a random direction. Over many absorption-emission cycles, the atom will gain a net momentum opposing the direction of propagation of the laser beam.
If an atom is moving toward or away from a laser beam, the frequency that it "sees" is up- or down-shifted, respectively, due to the Doppler effect. Thus, red-detuned laser beams will be shifted into resonance for atoms moving toward them. Those atoms will thus preferentially absorb photons from the laser beam opposing its direction of motion and will get net momentum kicks that slow it down and push it back toward the center. This can effectively cool atoms to several hundred micro-Kelvin and confine them to a volume that is given by the overlap volume of the laser beams.
To confine atoms even more and increase the density of the ultracold ensemble, a magnetic field in Anti-Helmholtz configuration is added. This magnetic field increases linearly with increasing distance from the center and vanishes at the center, and the atomic energy levels mimic this changing magnetic field (Zeeman effect) as shown in the figure below:
[1] E. L. Raab, M. Prentiss, A. Cable, S. Chu, and D. E. Pritchard, Trapping of neutral sodium atoms with radiation pressure, Phys. Rev. Lett. 59, 2631 (1987)
[2] S. Chu, The manipulation of neutral particles, Rev. Mod. Phys. 70, 685 (1997); C. Cohen-Tannoudji, Manipulating atoms with photons, Rev. Mod. Phys. 70, 707 (1997); W. D. Phillips,Laser cooling and trapping of neutral atoms, Rev. Mod. Phys. 70, 721 (1997)

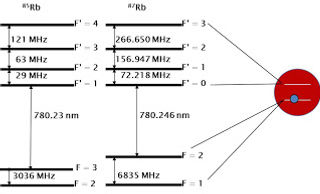
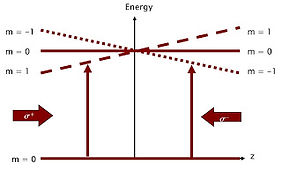
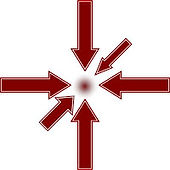